Thyristor diode, or silicon controlled rectifiers, have been an essential component in the world of electronics for decades. These powerful semiconductor devices have played a significant role in shaping the industry, enabling the development of various high voltage and high current applications. But have you ever wondered what makes thyristor diode so special? In this blog post, we will delve into the fascinating world of thyristor diode, revealing their unique characteristics, applications, and how they compare to other semiconductor devices.
As we journey through this comprehensive guide, you will uncover the intricacies of thyristor diode, including their structure, operational principles, and technologies. Furthermore, we will explore troubleshooting and maintenance techniques to ensure the safe and efficient operation of these devices. So, strap in and prepare to embark on an electrifying adventure as we unravel the mysteries of thyristor diode!
Key Takeaways
- Thyristor diodes are four-layer semiconductor devices used as controlled switches in high voltage and current applications.
- They can be tailored for various applications, including AC power control, high voltage protection, and relay replacement circuits.
- Troubleshooting and maintenance should be conducted regularly to ensure proper operation of the device within its Safe Operating Area (SOA).
Understanding Thyristor Diodes
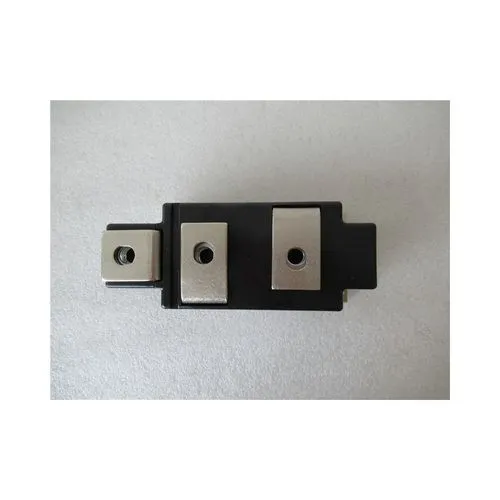
Thyristor diodes, also known as silicon controlled rectifiers (SCRs), are four-layer semiconductor devices with three terminals: anode, cathode, and gate. These devices function as controlled switches in high voltage and current applications. The gate signal, applied to the gate terminal, is responsible for controlling the silicon controlled rectifier and ultimately allowing current to flow.
Once triggered by a pulse at the gate terminal, the SCR will conduct current until the voltage between the cathode and anode is reversed or drops below a specific threshold or holding value. This unique characteristic offers several advantages, such as preventing simultaneous heat production in reverse conducting thyristors since the SCR and power diodes do not conduct at the same time, making them easier to integrate and cool.
Structure and Components
A thyristor diode’s behavior, especially regarding forward and reverse biased conditions, hinges upon its PNPN structure. This structure consists of alternating P-type and N-type semiconductor material with three PN junctions. In forward bias, the anode (P-region) is connected to a higher potential (voltage) than the cathode (N-region), while in reverse biased, the cathode is connected to a higher voltage than the anode, causing a voltage drop across the device. The PN junction, the boundary between the P and N-regions, plays a vital role in dictating current flow through the device when a forward voltage is applied.
Thyristor diodes come in two types distinguished by their leads: three-lead thyristors and two-lead thyristors. A small current controls the anode-to-cathode current in the three-lead thyristor. In contrast, the two-lead thyristor triggers conduction when a specific breakdown voltage is reached in the anode-to-cathode potential difference. This distinction highlights the versatility of thyristor diodes, as they can be tailored for various applications and requirements.
Operation Principles
A single positive current pulse applied to the gate terminal can trigger the conduction of thyristor diodes. The diode stays in the “ON” state until the anode-to-cathode current drops below the latching level necessary for latched operation. There are three primary modes of operation for a thyristor diode: forward blocking, forward conduction, and reverse blocking mode. The gate terminal serves a crucial function in initiating conduction by injecting a positive current, after which the thyristor will remain in conduction until the supply voltage is removed or the current falls below the minimum holding current.
For applications like power supplies demanding stable operation, the latching current is crucial. This is the minimal current necessary to maintain the thyristor in its on-state.
Grasping the operating principles of thyristor diodes equips engineers and technicians to harness their capabilities optimally in diverse applications. These devices offer precise control over current flow, making them invaluable components in numerous high voltage and high current situations.
Thyristor Diode Applications
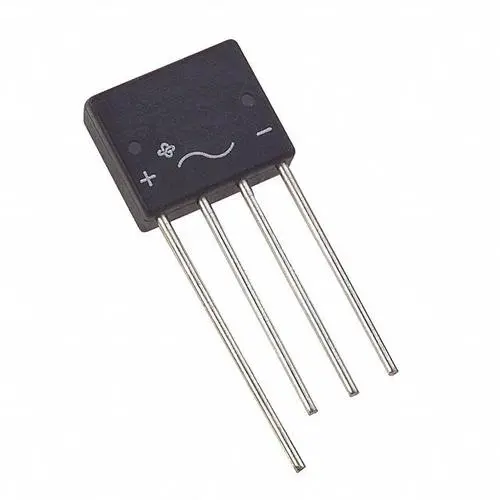
Applications of thyristor diodes span across a wide spectrum, including:
- Power-switching circuits
- Relay-replacement circuits
- Inverter circuits
- Oscillator circuits
One of their most notable uses is in AC power control, where they modulate the flow of current in an alternating current circuit through phase control. In forward blocking mode, the thyristor blocks the flow of current until a gate pulse is applied, allowing it to be precisely controlled.
Thyristor diodes serve a protective role in high voltage scenarios. They shield circuits from overvoltage and overcurrent conditions by limiting the voltage and current in a circuit, thereby averting potential damage to the components.
By examining the specific uses within AC power control and high voltage protection, one can truly appreciate the flexibility and potency of thyristor diodes.
AC Power Control
Various AC power control applications utilize thyristor diodes, including lighting dimmers, AC motor speed controls, and heaters. In these applications, the gate pulse is deployed to regulate the power output. Silicon controlled rectifiers are used in these applications to provide precise control of the power output by controlling the firing of the AC power. However, it is important to note that using TRIACs with heavily inductive motor loads can lead to the devices failing to turn off during the zero-voltage instants of the AC power cycle.
In conclusion, thyristor diodes are an indispensable component in AC power control applications, enabling regulation of the mean value of an AC load current without significant power dissipation. This makes them a popular choice for a wide range of industries and applications.
High Voltage Protection
In high voltage protection, thyristor diodes have a pivotal function. They discern high voltage levels and initiate preventative measures to avert circuit or device damage. In the event of an overvoltage situation, a thyristor diode can function as a circuit breaker, short-circuiting the power supply output to the ground and activating upstream circuit breakers or fuses to protect devices connected to the power supply. This protective action is known as the crowbar effect.
A crowbar protection circuit utilizes a thyristor diode in combination with a Zener diode connected to its gate. When the output voltage of the supply rises above the Zener voltage, the thyristor will conduct and short-circuit the power supply output to ground. This ensures that the power supply remains stable and prevents damage to connected devices, making thyristor diodes an essential component in high voltage protection applications.
Comparing Thyristor Diodes with Other Semiconductor Devices
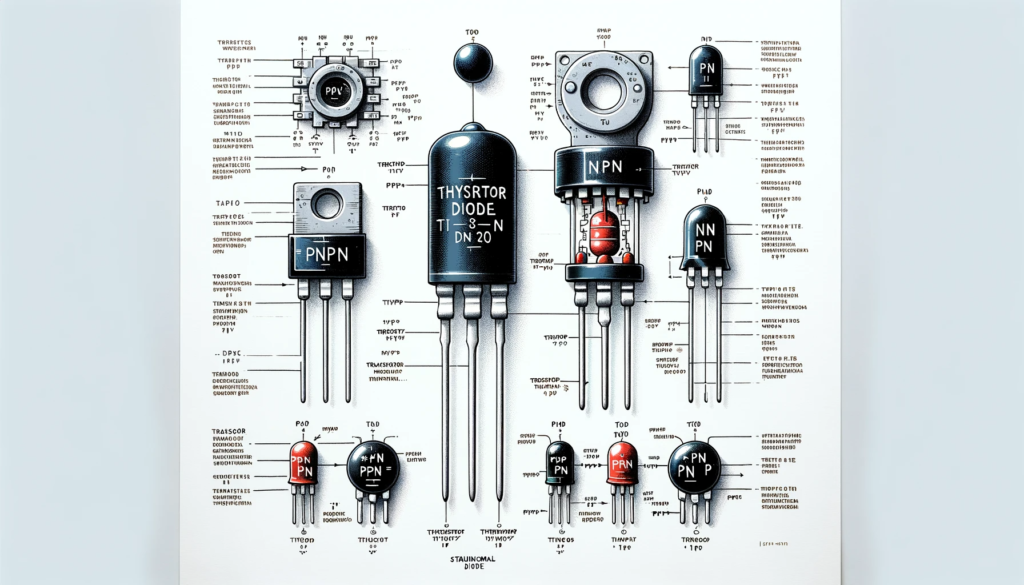
Though thyristor diodes have distinct characteristics and advantages, other semiconductor devices like transistors, IGBTs, and Power MOSFETs provide a variety of benefits and capabilities. Transistors, for example, can operate in a range of states and require a continuous signal to conduct current, whereas thyristor diodes can only be fully on or off. Despite their differences, these devices provide a variety of options for engineers and technicians when designing and optimizing electronic circuits.
The subsequent subsections will provide a deeper insight into the characteristics of transistors, IGBTs, and Power MOSFETs, emphasizing their commonalities and variances with thyristor diodes.
Transistors
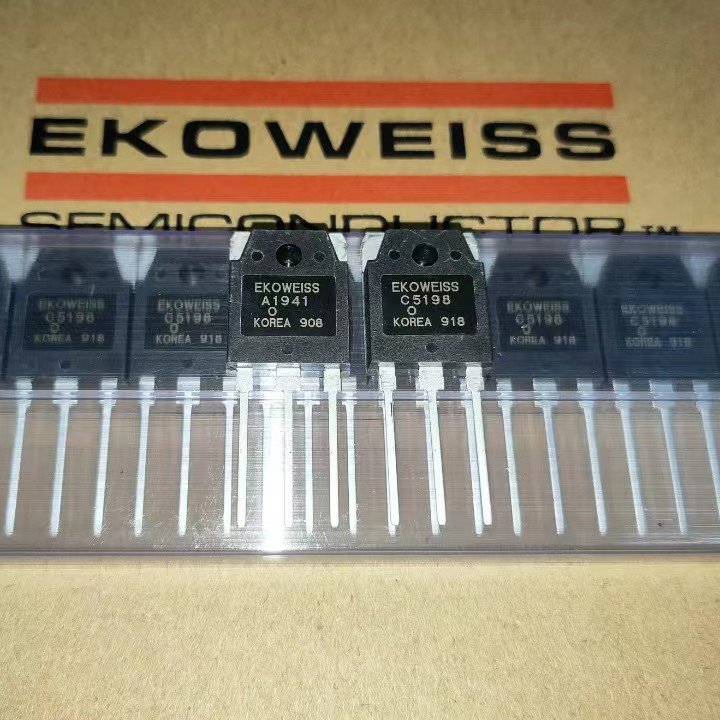
The waiting state of transistors sets them apart from thyristor diodes, as they require a continuously refreshed signal to conduct current. This characteristic allows transistors to operate more flexibly, as they can respond to a range of input signals and conditions. As a result, transistors are utilized in a variety of applications, often functioning as amplifiers and switches in electronic devices.
While transistors and thyristor diodes share some common features, such as the ability to control the flow of current, their operational principles differ significantly. Distinguishing these differences is of paramount importance when selecting the right device to meet a specific application or requirement.
IGBTs and Power MOSFETs
IGBTs and Power MOSFETs are semiconductor devices that offer superior switching characteristics compared to thyristor diodes. These devices are more commonly used in low to medium power applications due to their faster switching speeds, increased efficiency, and reduced power losses. As a result, IGBTs and Power MOSFETs have become popular choices for applications such as motor control, power conversion, and power supply circuits.
Despite their higher cost compared to thyristor diodes, the advantages offered by IGBTs and Power MOSFETs make them an attractive option for engineers and technicians seeking to optimize their electronic designs. Some of the advantages of these devices include:
- Higher switching speeds
- Lower power losses
- Better thermal performance
- Higher voltage ratings
- Improved efficiency
A thorough grasp of these devices’ strengths and weaknesses allows professionals to make knowledgeable decisions when choosing the most suitable semiconductor device for their applications.
Thyristor Diode Modules and Technologies
With the advancement in technology, a range of thyristor diode modules and technologies have emerged to meet specific application requirements. These modules and technologies offer unique features and benefits, ensuring that thyristor diodes remain a versatile and powerful solution for a wide range of applications.
The subsequent subsections will delve into two notable thyristor diode technologies: pressure contact technology and silicon carbide thyristors. As we examine each technology, we will gain a deeper understanding of their advantages and potential applications in the world of electronics.
Pressure Contact Technology
Pressure contact technology is used in thyristor diode modules to create a secure and reliable electrical contact between the components. This technology involves applying pressure to ensure maximum reliability, helping to prevent arcing and offering a short-on-fail feature for enhanced safety and performance. In Power Block modules, pressure contact technology is utilized to ensure the highest level of reliability.
The benefits of pressure contact technology make it an attractive option for high current applications in compact designs, while solder bond technology caters to performance-optimized applications. By understanding the advantages and drawbacks of each technology, engineers and technicians can select the most suitable option for their specific requirements.
Silicon Carbide Thyristors
Silicon carbide thyristors represent a significant advancement in semiconductor technology, offering improved performance in high-temperature environments up to 350°C. These devices utilize silicon carbide (SiC) as the semiconductor material, providing enhanced capabilities compared to traditional silicon-based thyristors.
Silicon carbide thyristors are ideal for high-temperature applications such as power electronics, motor control, and lighting control. Their higher switching speeds and temperature ratings, along with improved efficiency compared to power MOSFETs, make them an attractive option for engineers and technicians seeking to optimize their electronic designs.
Troubleshooting and Maintenance
Appropriate troubleshooting and maintenance are fundamental to guarantee the safe and efficient operation of thyristor diodes. Regular inspections should be conducted to maintain the cleanliness and dryness of the SCR and diode assemblies, and any dirt, dust, or other contaminants should be carefully removed using a soft cloth or brush. Additionally, devices such as stud-mounted SCRs and thyristors should be tested using a multimeter to measure the voltage across their terminals and assess their performance.
A clear comprehension of the troubleshooting and maintenance needs of thyristor diodes is key to increasing their reliability and lifespan. By adhering to proper maintenance practices, technicians and engineers can ensure that these devices continue to function optimally throughout their lifespan.
Safe Operating Area
The safe operating area of a thyristor diode is determined by the manufacturer, taking into account voltage, current, and power ratings to ensure device reliability. This area, known as the Safe Operating Area (SOA), indicates the voltage and current conditions in which a power semiconductor device can operate efficiently and without risk.
Compliance with the manufacturer-specified safe operating area is paramount to prevent device failure and ensure the reliable functioning of thyristor diodes. By staying within this range of voltage, current, and temperature values, engineers and technicians can optimize the performance of these devices and minimize the risk of damage or malfunction.
Snubber Circuits
Snubber circuits, consisting of capacitors and resistors, are used to limit the rate of voltage change in thyristor diodes, preventing unintentional triggering and ensuring reliable operation. These circuits can be connected in series or parallel, depending on the specific application and requirements of the external circuit.
Engineers and technicians can boost the reliability and efficiency of thyristor diodes and minimize the risk of overvoltage damage by integrating snubber circuits into their designs. Understanding the function and benefits of snubber circuits is an essential aspect of optimizing the performance of these powerful semiconductor devices.
Summary
Throughout this comprehensive guide, we have explored the fascinating world of thyristor diodes, uncovering their unique characteristics, applications, and how they compare to other semiconductor devices. We have delved into their structure and operational principles, as well as the various technologies and modules used to optimize their performance. Additionally, we have examined the importance of proper troubleshooting and maintenance for ensuring the safe and efficient operation of these devices.
As we conclude our journey into the world of thyristor diodes, we hope that you have gained a deeper appreciation for the power and versatility of these incredible semiconductor devices. With their myriad applications and potential for continued innovation, thyristor diodes are set to remain a vital component in the ever-evolving landscape of electronics.
Frequently Asked Questions
What is a thyristor diode?
A thyristor is a four-layer device composed of alternating P-type and N-type semiconductors, and it usually consists of three electrodes: an anode, a cathode and a gate. It is often used in conjunction with a Zener diode attached to its gate, and can be used to short-circuit the power supply output to ground when the voltage exceeds the Zener voltage. This device is useful for controlling the flow of current in a circuit, as it can be used to switch the current on and off. It can also be used to regulate the voltage in a circuit, as it can be used to limit the voltage to a certain level. This makes it a useful component
What is a thyristor used for?
A thyristor is an efficient and compact switch used to regulate electric power and current, capable of handling high voltages and currents up to 6000V and 4500A.
Can you use a thyristor as a diode?
Yes, a thyristor can be used as a diode because it has a unidirectional flow of current and can be used as an open-circuit switch or rectifying diode when its gate is triggered.
Why we use thyristor instead of diode?
Thyristors provide more control and accuracy in rectifier circuits than diodes due to the external triggering capability of the gate terminal, as well as a higher power handling ability. This makes thyristors the superior choice over diodes for many applications.
What are the three terminals of a thyristor diode?
The thyristor diode has three terminals: anode (A), cathode (C), and gate (G), which is used to control the device.