Microelectromechanical Systems (MEMS) are critical components in many technologies, shaping the functionality of everything from smartphones to vehicles. These microscale systems combine mechanical and electronic components to create sensors and actuators that improve our interaction with technology. Learn about MEMS’ role, their operation, and how they’ve become integral to so many aspects of modern tech.
Key Takeaways
- MEMS technology, comprising miniaturized mechanical and electrical components, has evolved since the 1960s and is now integral in industries such as consumer electronics and automotive, offering advanced sensors and actuators.
- MEMS fabrication is a precise and complex process influenced by integrated circuit techniques, utilizing materials like silicon and incorporating methods such as bulk and surface micromachining to create the microscale structures.
- The future of MEMS is promising, with potential growth in telecommunications, medical applications, and the integration with cutting-edge technologies like IoT, emphasizing the importance of addressing current manufacturing and design challenges.
Exploring the World of MEMS Technology
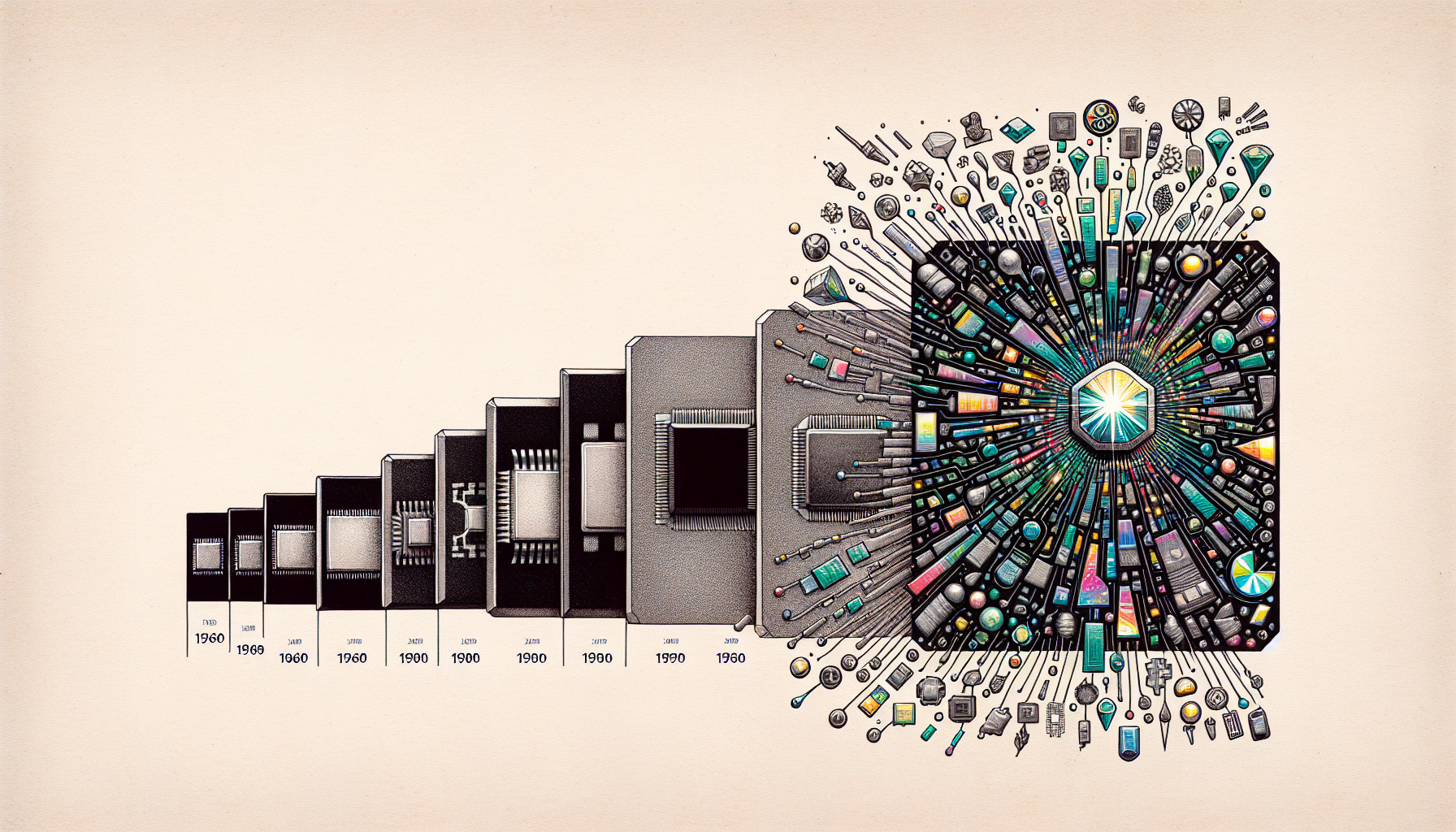
The journey of MEMS technology began in the 1960s and was partly inspired by Richard Feynman’s visionary concept of manipulating objects at a small scale. From the first batch fabricated surface micromachined MEMS device in 1964, MEMS technology has come a long way.
Nowadays, it’s prevalent in contemporary devices such as automobiles and smartphones, transforming how we interact with technology.
Defining MEMS and Their Role in Tech
MEMS represent devices that combine micro electro mechanical, electrical, and often optical components on a single microchip. These systems, also known as micro electro mechanical systems, are a subset of Microelectromechanical Systems. Also known as microsystems technology (MST) or micromachines, these devices have revolutionized various sectors, from consumer electronics to automotive safety, by enabling the creation of compact, efficient, and precise sensors and actuators.
The Historical Journey of MEMS Development
The 1960s marked the transition of MEMS from research laboratories to everyday products, highlighting a significant progression in their applications. A notable milestone in MEMS history was the development of the resonant gate transistor, the first batch fabricated surface micromachined MEMS device by Harvey Nathanson from Westinghouse. This innovation aimed to make micromachining of silicon compatible with planar integrated circuit technology, allowing for the integration of MEMS and integrated circuits on the same silicon wafer.
By the mid-1990s, MEMS components began to be integrated into a variety of commercial products, such as:
- force sensors for vehicle brakes
- blood pressure sensors
- inkjet printer heads
- fiber-optic components
This showcased the growing importance of MEMS in the technology sector, demonstrating their ability to transform industries and shape the future of innovation.
Delving into MEMS Device Components and Functionality
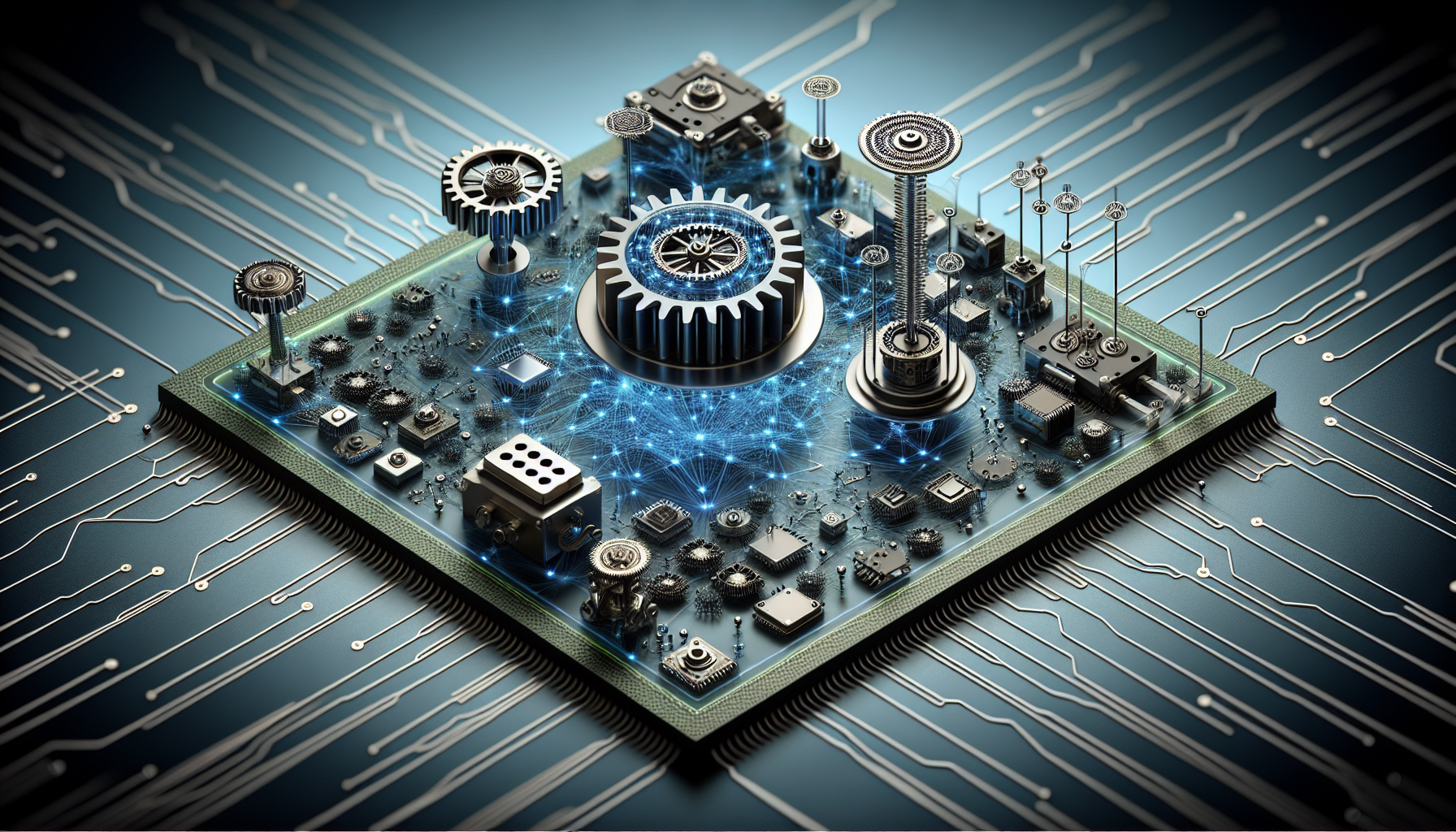
A MEMS device exemplifies a marvel of miniaturization, integrating mechanical microstructures, microsensors, micro-actuators, and micro-electronics onto the same chip. Materials common to MEMS devices include:
- silicon
- polymers
- metals
- ceramics
These materials are chosen for their unique properties and functionality.
These devices function as sensors or actuators, with integrated circuits providing an electrical interface, handling signal processing and compensation, and delivering an analog or digital output.
The Anatomy of a MEMS Device
Although a MEMS device may seem ordinary from the exterior, but delve deeper, and you’ll discover a world of complex microscopic structures. A MEMS device can include:
- channels
- holes
- cantilevers
- membranes
- cavities
All intricately designed and precisely manufactured. These devices range in size from as small as one micron to as large as one millimeter, showcasing their micro-scale design.
The functionality of MEMS devices springs from components like microsensors, microprocessors, and microactuators, each playing a critical role in the device’s performance. The sensors in MEMS devices are hybrid systems that include both mechanical elements for physical interaction and electronic components for sensing and data processing.
From Mechanical Signal to Electronic Response
A significant feature of MEMS devices is their ability to detect and measure mechanical signals, translating these into electrical responses. This is achieved by sensing changes in resistance or capacitance due to applied forces such as pressure. For instance, the core of a MEMS pressure sensor is comprised of a diaphragm and a fixed electrode. When pressure is applied, the diaphragm deflects, altering the capacitance and providing a measured mechanical signal.
This change in capacitance is then translated into an electrical signal, which can be processed and output as either an analog or digital signal. This makes MEMS devices particularly effective in applications where their compact size provides a distinct advantage over larger, conventional sensors.
The Art of MEMS Fabrication
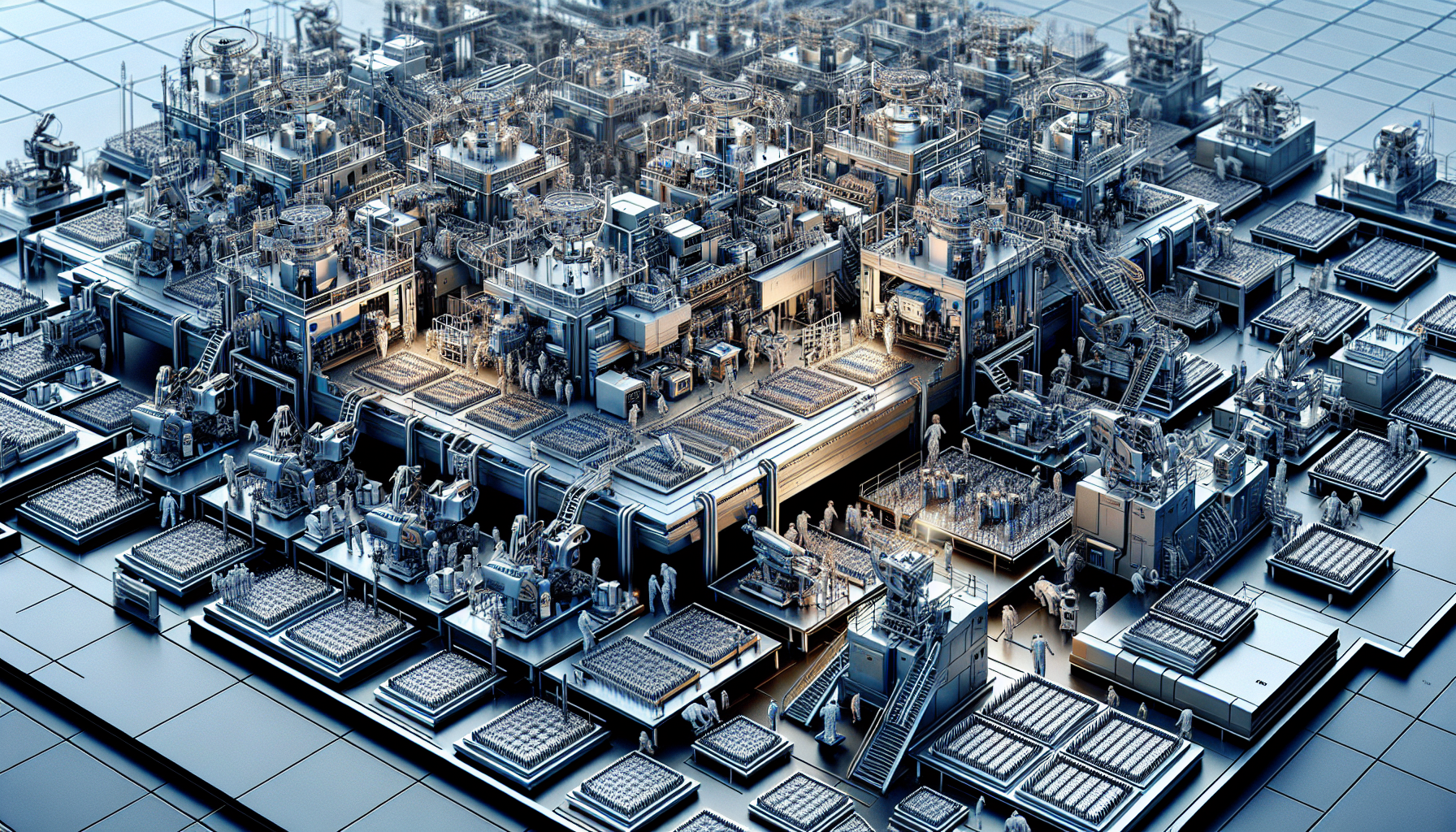
Creating MEMS devices is a highly intricate process that involves micro-fabrication technology, sharing similarities with batch processing methods used in integrated circuits production. This process includes additional steps like deep reactive ion etching and wafer bonding, tailored specifically for MEMS to create high aspect ratio features and combine wafers to form multi-layer structures.
Moreover, MEMS fabrication techniques encompass deposition of material layers, photolithography for patterning, and both wet and dry etching methods to achieve the desired microstructures, often utilizing same batch fabrication techniques.
Silicon Wafers and Thin Films: The Building Blocks
Silicon wafers form the core of MEMS fabrication, the fundamental component that provides the base material upon which MEMS devices are built. The orientation <100> of silicon wafers is often chosen for bulk micromachining of MEMS due to its wide availability and cost-effectiveness.
The economic success of MEMS devices hinges on the cost efficiency and ready availability of quality silicon wafers and thin film materials.
Precision Techniques: Bulk and Surface Micromachining
Precision is paramount in MEMS fabrication. Techniques such as:
- Bulk micromachining use silicon dioxide and silicon nitride as masking materials during isotropic wet etching.
- Anisotropic wet etching results in variable etch rates depending on the substrate’s crystallographic orientation.
- Creating thin silicon membranes with precise etch depths often involves the use of etch stops to ensure uniformity.
Specific unit processes, including deep reactive-ion etching and critical point drying, are employed in MEMS fabrication to achieve desired structural characteristics and prevent stiction. MEMS devices are mainly fabricated through surface micromachining, bulk micromachining, and molding processes.
MEMS Applications Across Industries
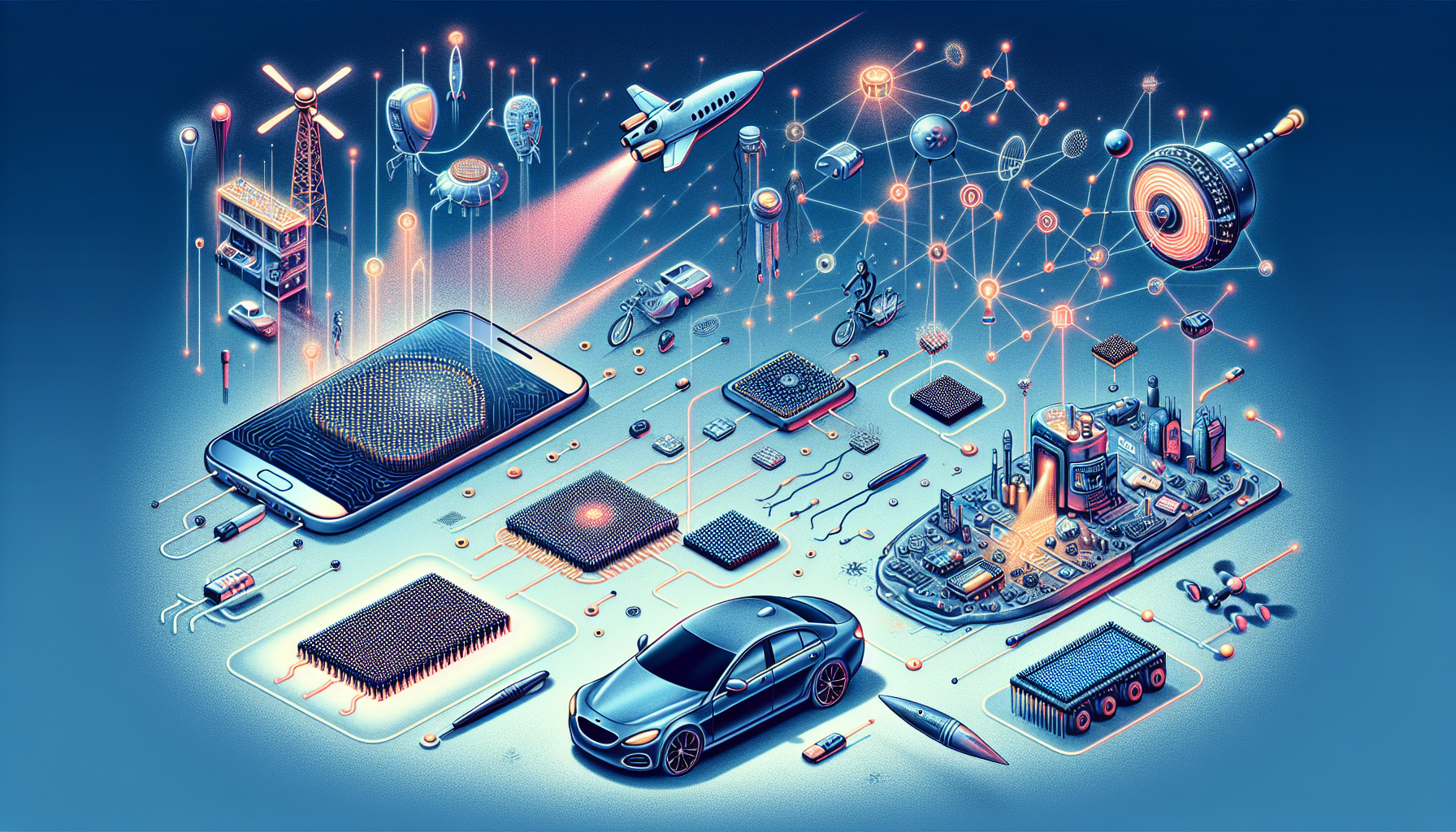
The adaptability of MEMS technology has contributed to its extensive application across various industries. From consumer electronics to automotive safety, MEMS sensors are a pivotal component, enabling features such as image stabilization, power management, and air quality monitoring. Accelerometers and gyroscopes in smartphones, MEMS microphones, and force sensors in vehicles are just a few examples of how MEMS components have become integrated into our everyday devices.
In the automotive sector, MEMS devices contribute significantly to safety, with applications in airbag deployment systems, tire pressure monitoring, and electronic stability control. The potential of MEMS technology extends even further, diversifying engineering product design and benefiting industries such as aerospace and defense.
Revolutionizing Consumer Electronics with MEMS Microphones and More
MEMS technology has significantly infiltrated the consumer electronics sector. Take smartphones, for example. Features like automatic screen orientation adjustment and image stabilization are all made possible by MEMS. And that’s not all. Smartwatches, with their ability to monitor various fitness activities such as walking and running, owe their practicality and market growth to MEMS sensors.
Beyond smartphones and smartwatches, MEMS sensors, including MEMS accelerometer, are enhancing user experiences in various ways, such as:
- Power management in hearables
- Multi-tap detection in hearables
- 3D audio through head tracking in hearables
- Motion control in gaming systems
- Gesture recognition in gaming systems
These applications offer a more interactive and immersive experience for users.
Even in laptops and tablets, MEMS sensors detect orientation and freefall events to activate protective measures for data. And let’s not forget MEMS microphones and sensors, which are increasingly integral to IoT products, bringing advantages such as small size, low power consumption, and manufacturability.
Enhancing Automotive Safety Through MEMS Sensors
Ensuring safety is vital in the automotive industry, and MEMS sensors play a crucial role in achieving it. Gyroscopes and accelerometers, for instance, are critical components in vehicle safety systems, enabling features like electronic stability control to function effectively. Since the 1990s, MEMS sensors have entirely replaced traditional mechanical crash sensors for airbag deployment in vehicles, showcasing their importance in automotive safety.
The automotive MEMS market is expected to experience a Compound Annual Growth Rate (CAGR) of 7%, reaching a valuation of $4.1 billion by 2028, largely due to the adoption of autonomous vehicles and advanced driver-assistance systems (ADAS).
The Synergy Between MEMS and Other Cutting-Edge Technologies
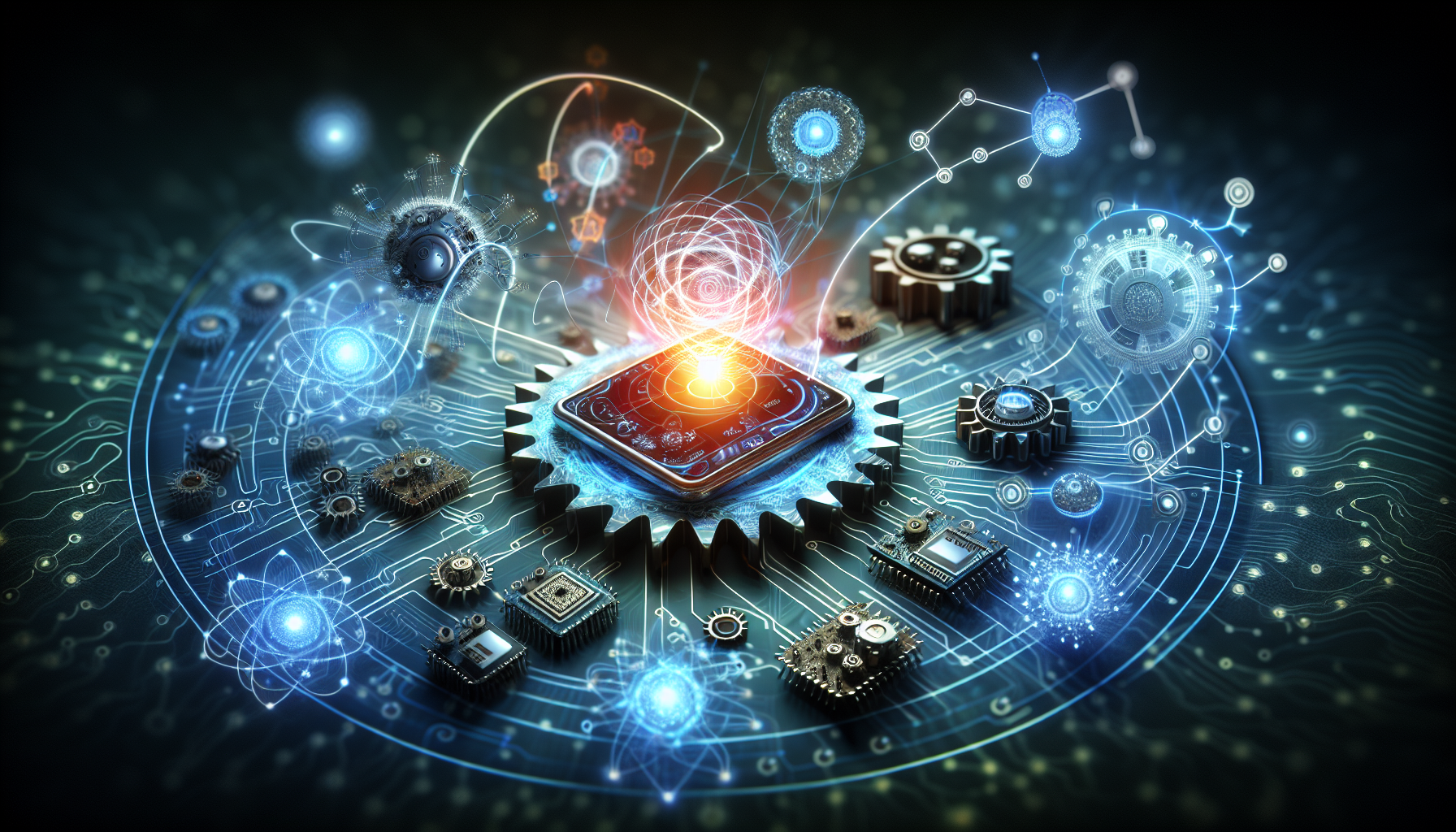
MEMS technology becomes even more potent when it synergizes with other cutting-edge technologies. For instance, in the realm of the Internet of Things (IoT), MEMS sensors and actuators are pivotal, allowing for the measurement of health metrics and data transmission to the cloud. The expansion of smart city concepts and artificial intelligence applications is expected to broaden the scope of MEMS sensor uses.
Even in the world of augmented and virtual reality, MEMS sensors are integral, tracking head movements to synchronize virtual images with the real world. The maturation of the IoT, along with artificial intelligence and autonomous driving technologies, is predicted to drive the future growth of the MEMS industry.
MEMS and IoT: Smarter Devices, Better Connectivity
Within the realm of IoT, MEMS sensors and actuators serve as more than mere components; they’re integral elements that enable the direct conversion of mechanical signals to electrical or optical ones. The integration of MEMS devices with microelectronics through surface micromachining could potentially reduce costs and improve the performance of IoT systems. Companies like STMicroelectronics have innovated intelligent sensor processing units (ISPU) that combine MEMS sensors with signal-processing and AI algorithms, resulting in efficient, local decision-making capabilities for IoT devices.
These ISPUs can significantly lower power consumption by up to 80%, offering enhanced efficiency for activities such as activity recognition and anomaly detection in smart devices. Smart home devices utilize MEMS sensors to monitor environmental parameters such as air quality and humidity, as well as conserving power through automatic wake-up and sleep functions.
In the realm of health and fitness, MEMS technology in IoT smartwatches facilitates the tracking of health-related metrics including:
- heartbeat rate
- blood pressure
- calorie burn
- step count
with seamless data transfer to the cloud. MEMS microphones from Knowles, suitable for IoT applications, offer a variety of options in size and performance, including sophisticated voice-trigger features that enhance device interactivity.
An AI-enabled true wireless stereo (TWS) development platform from Knowles employs integrated MEMS microphone arrays and speaker driver assemblies, elevating audio applications within IoT devices.
MEMS Meet Nanotechnology: Scaling Down to Atomic Levels
Delving further into the microscopic realm, we encounter the intersection of MEMS and nanotechnology. This union leads to applications in diverse fields, including:
- Biotechnology: MEMS and Nanotechnology enable advancements like DNA amplification systems, biochips for detecting hazardous agents, and microsystems for drug screening.
- Medicine: MEMS pressure sensors have been widely adopted in medical applications for monitoring blood pressure, intrauterine pressure during childbirth, and vitals in respiratory monitoring.
- Communications: MEMS and Nanotechnology play a crucial role in the development of miniaturized devices for wireless communication.
- Inertial sensing: MEMS accelerometers and gyroscopes are used in various applications, such as navigation systems, gaming consoles, and virtual reality devices.
These are just a few examples of the wide range of applications that MEMS and nanotechnology can enable.
In the communications sector, RF-MEMS technology enhances high frequency circuits by improving components such as inductors and capacitors, leading to better performance and lower costs. MEMS inertial sensors, like accelerometers and gyroscopes, have replaced traditional sensors in automotive air-bag deployment systems and are now used in consumer electronics.
Addressing the Challenges in MEMS Manufacturing and Design
Even with the substantial progress and extensive applications of MEMS, the technology faces challenges in design and fabrication. The challenges include:
- The process of miniaturization and integration of mechanical and electronic components present significant complexities.
- Optimizing the mechanical properties of materials used in MEMS is crucial for device performance and durability.
- MEMS packaging at the wafer level is complex, with difficulties arising from testing each die on the wafer, handling, and wire bonding spacing issues.
Material Selection and Mechanical Properties
The mechanical properties and dependability of MEMS devices are heavily influenced by the material chosen, especially when deployed in harsh environmental conditions. Thin films used within MEMS devices exhibit mechanical properties that can greatly vary from the bulk material, owing to differences in composition, microstructure, and manufacturing techniques. Mechanical properties such as residual stress and Young’s modulus are crucial for the performance of MEMS devices and are affected by settings in the fabrication process.
The development of international standards for assessing the mechanical properties of materials used in MEMS supports advancements in the reliability of MEMS technologies. Materials such as polysilicon, widely studied and employed in MEMS technology for its mechanical properties, and silicon carbide, recognized for its high strength and stiffness, showcase the considerations necessary for material selection in MEMS devices.
Overcoming Design Complexities
As more functions are incorporated into MEMS devices, their design complexity escalates. Advanced lithography techniques, such as electron beam lithography, are crucial for addressing these complexities. By beating the diffraction limit of light, electron beam lithography allows for more precise and complex MEMS designs necessary for multi-function integration.
These advanced techniques are key to addressing the complexities associated with integrating multiple functions into a single MEMS device.
The Future Landscape of MEMS Innovation
MEMS technology harbors a future rife with potential. Potential medical applications of MEMS include lab-on-a-chip devices for diagnostics and implantable devices for real-time monitoring of patient health. Innovations in MEMS technology could revolutionize personalized medicine by empowering implantable and wearable sensors that track individual health metrics.
Research in MEMS is looking toward developing even more advanced systems, enabling greater integration and complexity within the same small form factor. Future MEMS technology may offer improved performance through new materials and designs, enhancing sensitivity, accuracy, and functionality.
Pioneering Medical Devices with MEMS
The integration of MEMS devices into medical equipment is on the rise as key components that facilitate better and more cost-effective diagnostics. MEMS technology has a wide range of biomedical applications, including:
- Advanced drug delivery systems
- Implantable sensors
- Lab-on-a-chip and microfluidic devices
- Pressure sensors for monitoring purposes, such as monitoring blood pressure and intrauterine pressure during childbirth.
In addition to these applications, innovations in MEMS technology are enabling the development of:
- Bio-injectible devices that monitor health indicators
- Bio-implantable devices that monitor health indicators
- Bio-ingestible devices that monitor health indicators
As the trend of diagnostic and monitoring equipment miniaturization continues and the proliferation of wearable medical devices increases, the medical market for MEMS is projected to grow significantly.
MEMS technology is enhancing medical devices by adding sensory capabilities to minimally invasive tools, allowing unobtrusive monitoring and personalizing medicine.
MEMS in the Next Decade: What Lies Ahead?
Looking ahead, the telecommunications market is anticipated to experience the highest growth rate for MEMS applications in the coming decade. Within the telecom sector, optical MEMS for switching, MEMS oscillators, and MEMS switches will be key in addressing the increasing demand for data.
As MEMS technology continues to evolve, original equipment manufacturers (OEMs) will focus on optimizing costs, size, and performance, leading to new technologies and broader applications.
Summary
In a world where devices are becoming increasingly smaller and smarter, MEMS technology stands out as a key enabler. From their inception in the 1960s to their widespread application across industries today, MEMS have revolutionized our daily lives. As we move towards a future where technology continues to evolve at an unprecedented pace, the role of MEMS is set to become even more pivotal. Whether it’s in the latest smartphone, a life-saving medical device, or an autonomous vehicle, MEMS technology will continue to drive innovation, enhance our experiences, and shape the future of technology.
Frequently Asked Questions
What is a MEMS device?
A MEMS device is a miniature machine with both mechanical and electronic components, typically spanning from several millimeters to less than one micrometer in size.
What is a MEMS device made of?
A MEMS device is made of mechanical microstructures, microsensors, micro-actuators, and micro-electronics integrated onto the same chip. The most common materials used are silicon, polymers, metals, and ceramics.
How does a MEMS sensor work?
A MEMS sensor works by detecting mechanical signals through changes in resistance or capacitance caused by applied forces like pressure. It then converts these variations into electrical signals for processing and output.
What are some applications of MEMS technology?
MEMS technology has diverse applications, including image stabilization and air quality monitoring in consumer electronics, safety features in automotive systems, and sensor and actuator use in IoT devices for health data measurement and cloud data transmission. These applications highlight the expansive reach and impact of MEMS technology in various industries.
What are some challenges in MEMS design and fabrication?
In conclusion, challenges in MEMS design and fabrication include miniaturization, integration of components, and optimizing material properties. Wafer-level packaging poses complexities related to testing, handling, and wire bonding spacing.