The heart of modern electronics is the digital transistor that is accelerating all this progress and changing the way people live, work, and communicate. Those tiny components are fundamental to almost every electronic device, from your cellphone or laptop computer to the complex systems in servers, supercomputers, and advanced artificial intelligence platforms, which could not work without them. This is a definitive guide on how digital transistors bring electronics to be faster, smaller, and smarter in redesigning industries and unleashing pathways to hitherto dreamed-of items from science fiction.
This guide examines the technology behind the digital transistor, its influence on device performance, and how future trends shape this dynamic field.
1. Introduction to Digital Transistors:
A digital transistor is a semiconductor apparatus that operates fundamentally like an electronic switch, controlling the flow of electrical current within circuits. It is the basic building block of digital logic, which forms the basis of computing. Unlike analog transistors, which handle continuously varying signals, digital transistors operate with discrete on (1) and off (0) states and are, therefore, best suited for digital electronics.
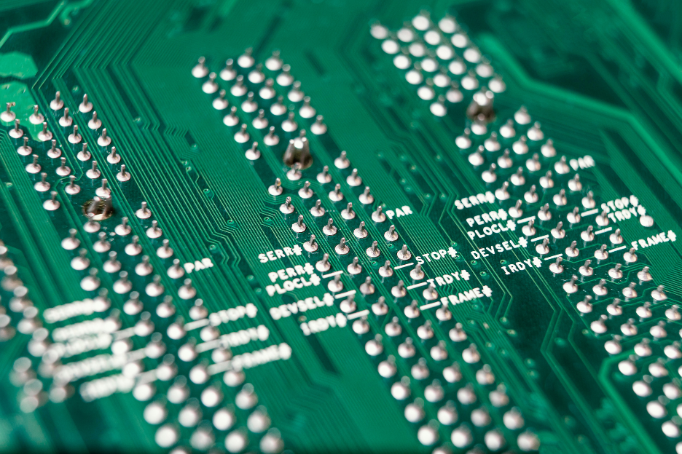
Structure and Operation: The basic building blocks of a digital transistor are three: the gate, the source, and the drain. When there is an applied voltage at the gate, the transistor acts to control the flow of current between the source and the drain. These components are determined by the choice of the material used in their construction, the most common being silicon.
The most common ones are MOSFETs, BJTs, and IGBTs. Each type is unique, having some characteristics depending upon the application, so some may seem ideal for high-speed switching and less power consumption, while others are good for high current handling.
2. Acceleration of Electronics:
Speed Revolution through Digital Transistors
One of the major ways digital transistors have changed electronics is in the fact that they greatly increase the speed of devices. This translates into faster transistors that imply quicker data processing, faster latency, and better performance as a whole.
High-Speed Switching: Digital transistors, more specifically MOSFETs, have the highest switching speed in comparison with all others. The development rate of switch-on or switch-off at extremely high frequencies with an advancement of technology improves the processors to execute billions of instructions in one second. This is very important when speed becomes a critical parameter in applications such as data centres and gaming.
Lower capacitance and resistance: The switching speed depends on the internal capacitance and resistance of a transistor. Modern transistors do not have parasitic capacitance and resistance, which enhance the mobility of electrons and cause the transistor to switch to its new state quickly. It is therefore this reduction in delay that fully accounts for the increase in modern processor speeds.
Material Innovation: Beyond Silicon, Gallium Nitride (GaN), and Silicon Carbide (SiC): Newer materials move way ahead of silicon and have attracted attention for further speed increases in digital transistors. These materials have higher electron mobility, can withstand operation at higher voltages and temperatures, and are suitable for high-speed applications.
Scaling and Miniaturization: As transistors are scaled down, fewer electrons travel the distance to propagate the signal. That means the propagation delay goes down. Further miniaturisation—the progression from 14 nm to 7 nm and now 3 nm processes—improves switching speed and thereby performance speed.
3. Electronics Miniaturization:
Transistor Scaling as a Determinant of Reduced Electronic Component Size The miniaturisation of digital transistors has been one of the significant determinants for the diminutive size of electronic components so that they become portable and efficient as well. More components can be supported in a smaller chip by smaller transistors-a powerful, compact device.
Scaling was something Moore described, which sets the pace for semiconductors’ doubling every two years on the number of transistors. Known as Moore’s Law, this prophecy allows scaling from transistors to date that has led to the creation of chips with billions of transistors that power everything from smartphones to supercomputers.
FinFET and Beyond: The greatest stride of miniaturisation would be from the traditional planar transistor to a FinFET. FinFETs utilize a 3D shape, which provides much better current control as well as reduced leakage currents as the scale decreases. GAA and nanosheet transistors that shrink even further follow these innovations.
SoC integration: In the SoC, several components such as CPUs, GPUs, and memory reside within one chip. Electronic devices therefore have a smaller footprint overall. All this is assisted by constant size reductions in transistors, thereby allowing high functionality on minimal form factors.
Impact on Mobile Devices: It has primarily been experienced in mobile devices and remains one of the most important drivers of the slimmer designs seen in improved performance and longer battery life in mobile devices. From foldable smartphones to wearables, transistor reduction has been a key enabler of new form factors and features.
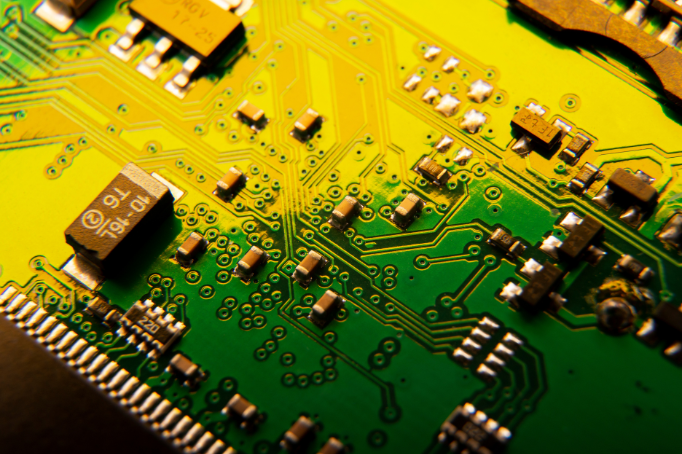
4. Smart Electronics: Add Functionality with Digital Transistors:
New digital transistors make electronics both faster and much smaller, truly smarter: the sense of intelligence behind them comes more directly from the ability of modern transistors to perform complex computations, process large amounts of information, and support sophisticated algorithms.
AI and Machine Learning: Artificial intelligence and machine learning represent new demands for transistor technology. Advanced transistors enable large neural networks and AI models not to battle with the computational performance that’s required to implement applications like speech recognition, computer vision, and predictive analytics.
Power Efficiency: More intelligent electronics will require smart power management, particularly for portable and battery-operated applications. Digital transistors are introduced with designs that should limit the consumption of power through low-voltage operation and energy-efficient switching. The techniques of dynamic voltage scaling as well as power gating will be included to enhance efficiency.
Error correction and reliability: modern transistors are equipped with an error correction ability, thereby increasing the reliability of their performances even when exposed to challenging conditions. Most applications, such as the making of autonomous cars and medical appliances must ensure precision and safety.
Sensor Integration and IoT: Digital transistors are central in the increasingly expanding world of the Internet of Things (IoT). Sensors and devices talk to each other to make smart environments. Transistors enable this seamless integration of sensors, actuators, and processors in real time for processing and decision-making.
5. History of digital transistor design:
Semantic richness in innovations pertinent to the semiconductor industry has continued to drive improvements over the years in digital transistor design with higher performance, efficiency, and functionality.
The most important advancements to date in terms of the design of transistors are from being planar to 3D transistors, including FinFETs and Gate-All-Around. This design achieved better control of current flow with lower leakage and overall improved performance at smaller scales.
New Materials and Fabrication Techniques To break free from the physical bound of the old silicon transistors, there are promising alternatives in graphene, carbon nanotubes, and transition metal dichalcogenides, materials whose electrical characteristics are not only better but also potentially to be used as substitutions or supplements to silicon.
Quantum and spin-based transistors. The new electronics revolution may be introduced by quantum transistors and spin-based devices that exploit the quantum world and even electron spin to perform operations at speeds and efficiencies far beyond anything achievable with current technologies.
Advanced Lithography: The EUV lithography has further promoted the transistor manufacturing technique toward even more precise and smaller components. This is indeed where this advanced fabrication method is destined to play a vital role in sustaining the transistor miniaturisation pace.
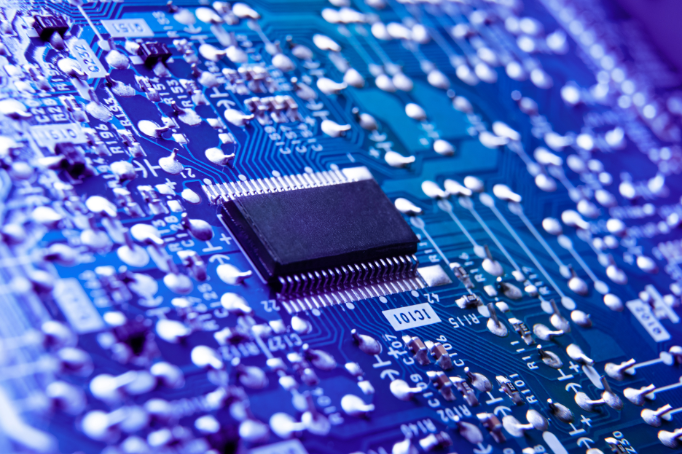
6. Obstacles in the Invention of Digital Transistors:
Digital transistors have made tremendous strides, but challenges crop up that are likely to become huge obstacles in the future of development.
Heat Management: As transistors shrink and speeds rise, it becomes complicated to keep the flow of heat dissipation intact. Heat dissipation can also cause degradation in performance and reliability, thus requiring better cooling solutions and methods for thermal management.
Power leakage is one of the greatest challenges, particularly at the lower scales. Leakage currents waste power and, more seriously, erode the overall stability of circuits. New designs, as well as novel materials, are being developed to fight this phenomenon.
Quantum Effects: Quantum effects, due to tunnelling and noise, can disrupt normal transistor behaviour at nanoscale dimensions. New design and material science approaches are required to address these effects.
Manufacturing Complexity Because of their high sophistication, manufacturing complexity and costs are introduced. As the number of iterations to reach the desired scale of transistors increases, the expense of manufacturing can become economically and technically unmanageable for the industry.
7. Transistors: Future of Digital Devices:
Digital transistors hold great promise for the future; several trends and technologies hold promise for the future to come.
Scaling Beyond Silicon: Even though silicon transistors have hit limits, the industry is not looking at new materials and architectures to continue at the same pace. The domain of work is in two-dimensional materials, quantum dots, and neuromorphic computing. Presently being actively researched are two-dimensional materials, quantum dots, and neuromorphic computing.
Integration with AI: Such specific transistors shall be used in AI applications such as tensor processing units and neuromorphic chips. Those specialized transistors optimize complex computations required by AI algorithms in the next intelligent electronics wave.
Flexible and Wearable Electronics: Advances in flexible and organic transistors allow for the development of wearable electronics and bendable screens, along with other novel form factors that will alter the landscape of consumer electronics.
Energy Harvesting and Low-Power Designs: Future transistors will be exhaustively energy-conscious, with designs that minimise power consumption.
Conclusion:
The digital transistors are changing the electronics market. Customers now gain the opportunity to find their products to become faster, smaller, and more intelligent with the advancements being driven. The small components, once equipped with higher efficiency, allow devices to process data at super-fast rates and therefore can execute more demanding applications like AI algorithms for high-speed communications. It reduces not only the portability but also enables innovations to the scale that help in wearables, IoT devices, and smart technology. In addition, the development of wiser transistors is driving progress in wiser systems and everything from more personal gizmos to industrial-style applications on large scales. Further research in transistor technology will further illuminate the manifestation of electronics that are faster, much more compact, and more intelligent than those currently realized.