Semiconductors power the modern digital era, but what raw materials are needed for semiconductors to create these technological cornerstones? This article delves straight into the essential materials of semiconductor manufacturing: silicon, germanium, and gallium arsenide, explaining their roles and why these materials are irreplaceable in the industry. Discover the significance and functions of these raw materials as we explore the backbone of electronic devices and future innovations.
Key Takeaways
- Semiconductors are built using materials like silicon, germanium, and gallium arsenide, each offering unique properties such as electrical conductivity, efficiency, and heat resistance. Doping agents like boron and phosphorus are critical for altering electrical properties and enhancing performance.
- Advanced materials such as carbon nanotubes and nanomagnetic chips present opportunities for improving energy efficiency and speed in semiconductor technology, positioning them as potential successors to traditional materials like silicon.
- The semiconductor manufacturing process is complex and multifaceted, involving steps such as wafer creation, photolithography, and etching, and facing challenges in materials handling, sustainability, and innovation to meet the increasing demands and applications in various industries.
Key Ingredients in Semiconductor Fabrication
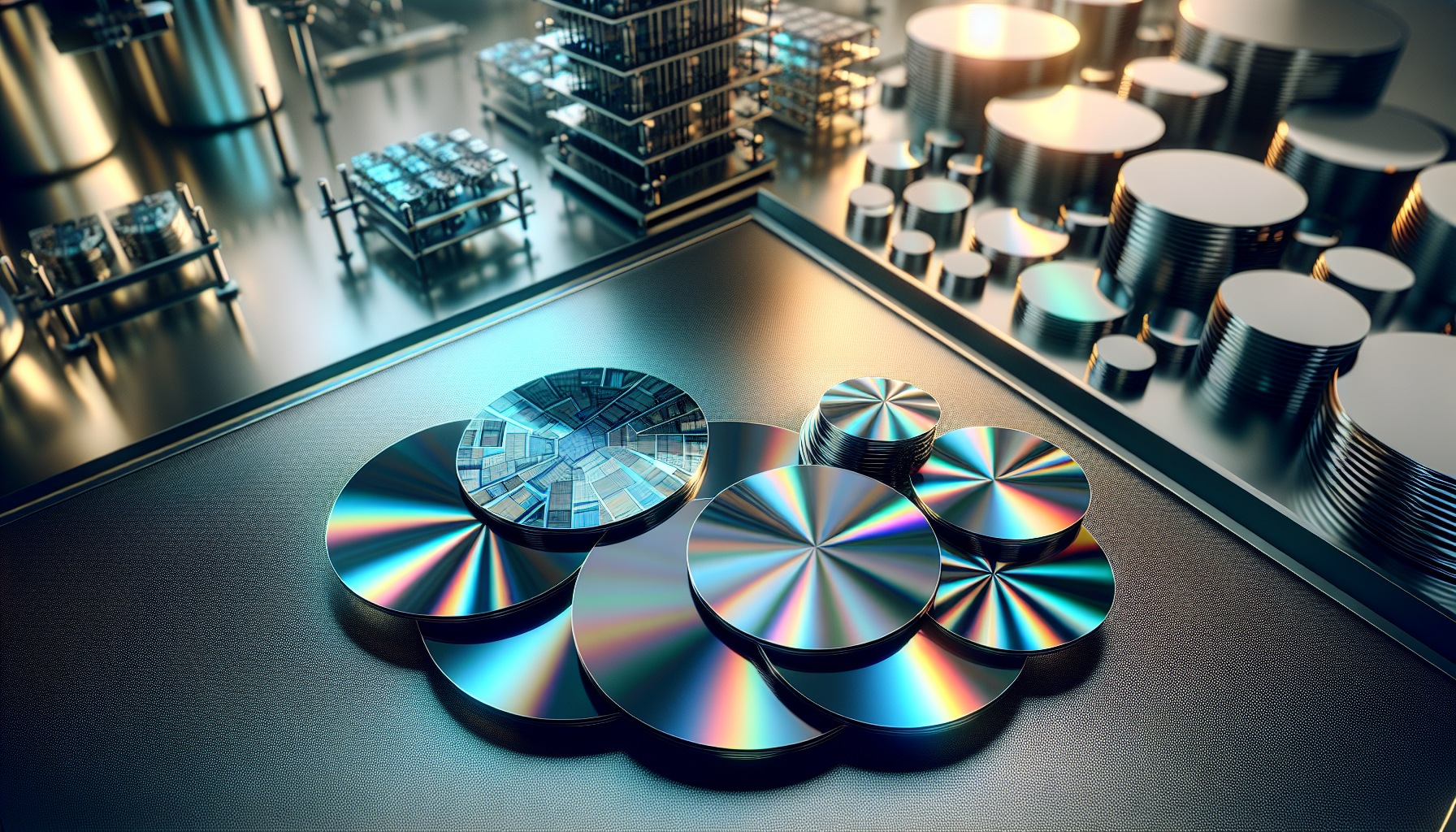
Semiconductors are the heart of our electronic world. But what gives life to these microscopic wonders? The answer lies in the raw materials used in their fabrication, each playing a unique, instrumental role. Silicon, germanium, and gallium arsenide are the key ingredients in the manufacture of semiconductors, each providing a distinct set of properties that make them indispensable in the semiconductor industry.
Silicon, the bedrock of electronics, is the most commonly used material, known for its exceptional electrical properties. Germanium, although less common, plays a significant role in the creation of high-efficiency devices such as solar cells and infrared detectors. On the other hand, gallium arsenide is selected for its high-temperature tolerance, making it ideal for devices that operate at elevated temperatures.
Silicon: The Bedrock of Electronics
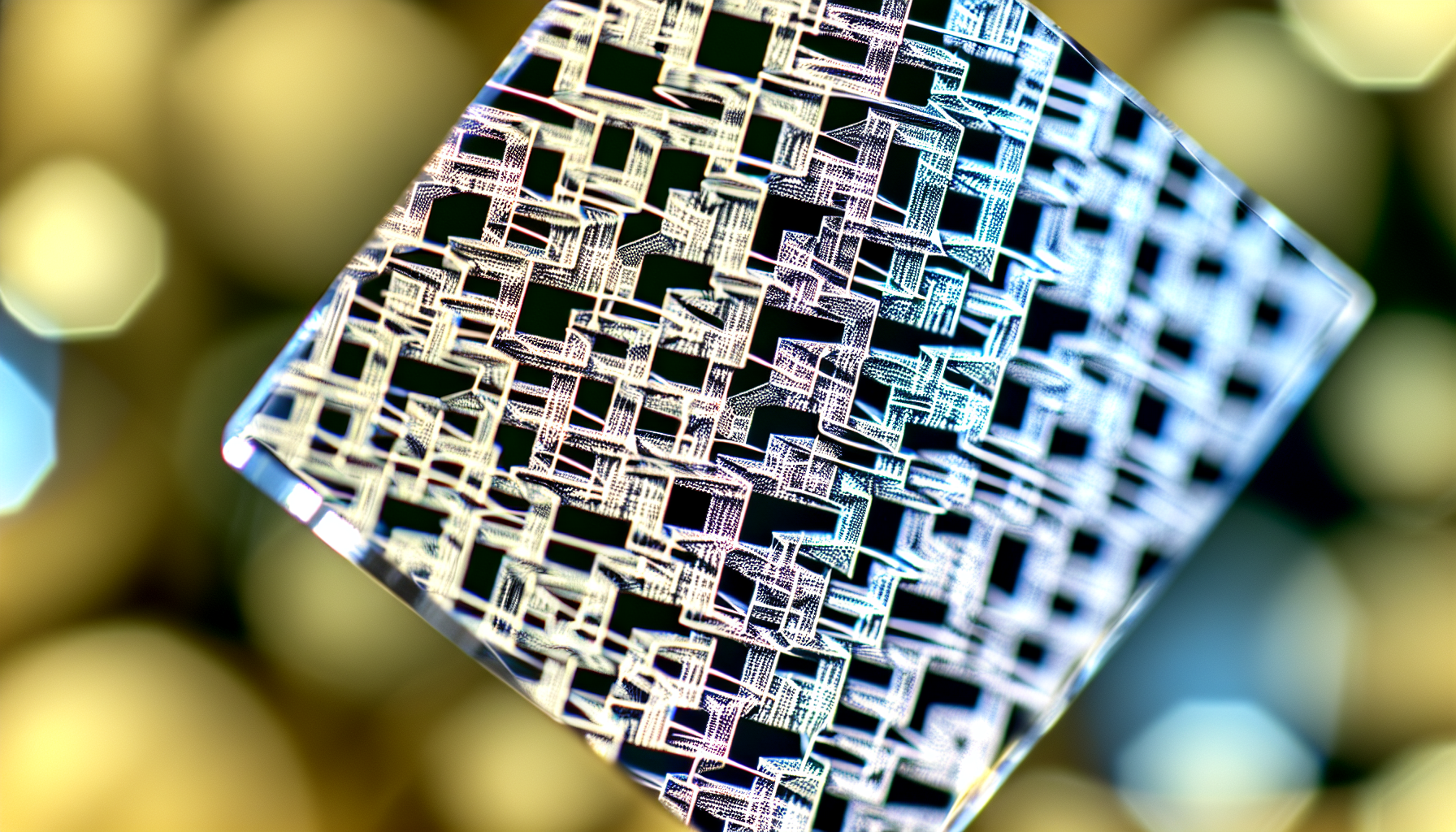
The most widely used semiconductor material in the sector is Silicon, thanks to its stable structure and remarkable properties, including the ability to generate high-quality insulating oxide layers. Consequently, it serves as the principal material for transistors in electronic circuits and devices.
The transformation of raw silicon into silicon wafers for semiconductor manufacturing involves a complex process that can take more than three months. This includes the purification of silica sand and the growth of silicon crystals using the Czochralski method. However, the use of silicon in computer chip manufacturing is not without its challenges. Its performance tends to degrade at elevated temperatures, which is a limitation that alternatives like GaN semiconductors do not face. Yet, the manufacturing process for such alternatives may be more expensive than that for silicon, giving rise to economic considerations.
Germanium’s Role in High-Efficiency Devices
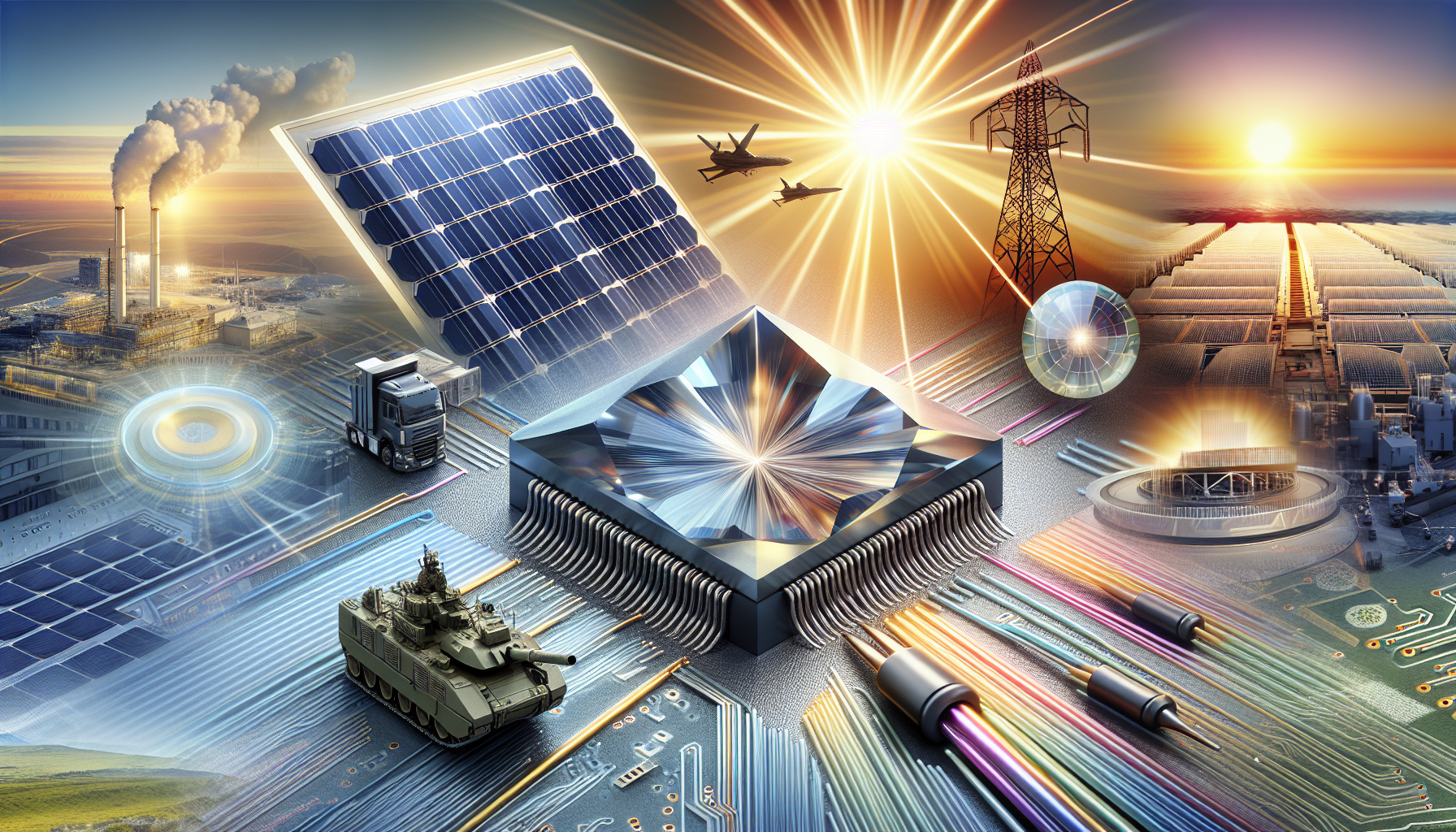
Germanium, a rare semiconductor, is renowned for its high-speed electronic properties. Its unique characteristics render it suitable for high-efficiency devices, such as the production of efficient electronic and optoelectronic devices. One of the techniques used for introducing dopants into germanium-based semiconductors is ion implantation.
The role of Germanium extends to solar cell manufacturing, where it serves as a growth template, improving the performance of specific cells. Furthermore, in the realm of high-speed communications, germanium is used to regulate the index of refraction of optical fibers, facilitating rapid data transmission through light pulses. While its incorporation into semiconductor fabrication presents certain challenges, its potential benefits cannot be overlooked.
Gallium Arsenide: For High-Temperature Tolerance
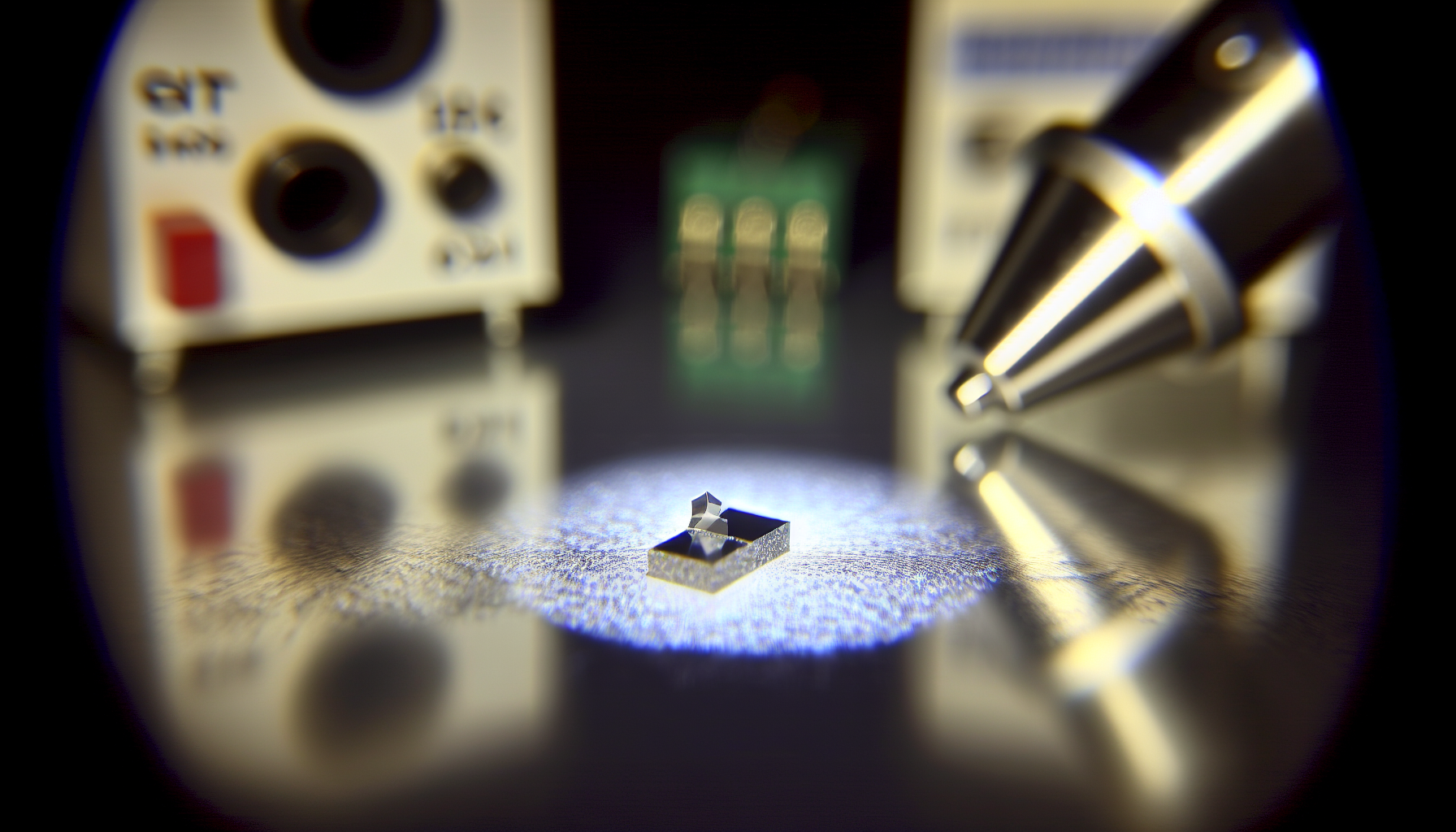
For applications requiring high temperatures, gallium arsenide emerges as the preferred material. Its wide band gap, high dielectric constant, and excellent thermal stability make it an ideal candidate for thin films in microwave circuits, infrared lights, and optical devices.
Moreover, gallium arsenide devices are capable of operating at very high frequencies, reaching 100 GHz and beyond. This makes them well-suited for high-speed applications, such as:
- semiconductors
- microwave frequency circuits
- solar cells
- lasers
- sensors
- detectors
- communication systems
- optoelectronics
This cements gallium arsenide’s position in the electronic applications landscape.
Doping Agents: The Conductivity Enhancers
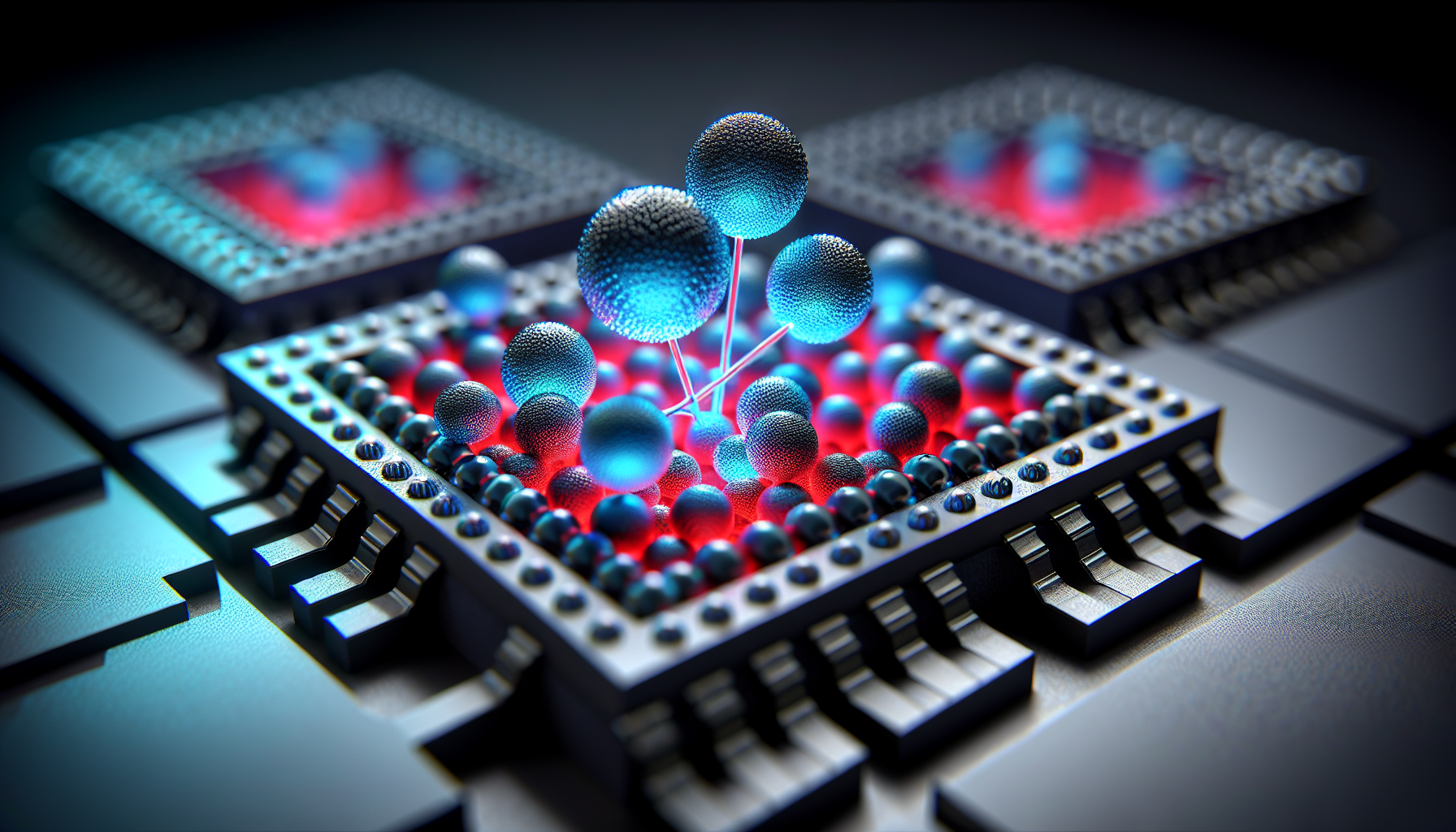
Just as semiconductors are the heart of electronic devices, doping agents could be considered the lifeblood. These are impurities intentionally introduced to alter the electrical conductivity of the semiconductor crystal, thereby enhancing their performance. Some common doping agents include:
- Boron
- Phosphorus
- Arsenic
- Antimony
- Gallium
These doping agents play a crucial role in the functioning of various electronic devices.
Two such agents, boron and phosphorus, play a crucial role in the creation of p-type and n-type semiconductors, respectively. Boron increases the conductivity of semiconductors by inducing ‘holes’ in the structure, leading to the development of a p-type semiconductor. Phosphorus, on the other hand, augments the conductivity of semiconductors through the donation of an additional electron, leading to increased conductivity and the formation of an n-type semiconductor.
Boron – P Type Semiconductor’s Best Friend
Boron, a metalloid, plays an essential role in enhancing the performance of computer chips and making insulators more conducive to electricity. It is employed as a dopant in p-type semiconductors, introducing trivalent impurities. This results in a shortage of electrons, or ‘holes’, within the structure of the semiconductor, generating positive charge carriers that facilitate electrical conductivity.
Furthermore, boron improves the performance of computer chips by utilizing its compound, boron arsenide. This enables the chips to manage increased operational power by enhancing the dissipation of heat from hotspots and maintaining high conductivity, thereby supporting more efficient chip function.
Phosphorus – The N Type Semiconductor Contributor
Phosphorus, a key dopant in semiconductor chips, enhances the conductivity of semiconductors by:
- Replacing silicon atoms and freeing up electrons for improved conductivity
- Introducing additional electrons into the silicon crystal structure when it is doped with silicon
- Functioning as mobile charge carriers, thereby augmenting the semiconductor’s conductivity.
The process of doping semiconductors with phosphorus entails the introduction of phosphorus impurities into a semiconductor crystal to alter its electrical properties. However, this process is not without its challenges such as the condensation of phosphorus vapor, which can result in pollution and fire hazards. Therefore, selective doping techniques are necessary to prevent unwanted contamination when exposing the entire semiconductor surface during doping.
Metals and Insulators: Completing the Circuit
Aside from key materials and doping agents, metals and insulators are other vital elements that complete the circuitry in semiconductor devices. Metals, such as copper, are used in the fabrication of semiconductor devices due to their lower resistance in comparison to other metals like aluminum, resulting in the production of quicker microprocessors. On the other hand, insulators play an integral part by providing protection against high voltages and preventing electrical shorts between cables.
Apart from copper, semiconductor circuits frequently integrate metals like aluminum, gold, and tungsten, with each metal offering distinct properties that contribute to the device’s performance. Simultaneously, insulators are of utmost importance in semiconductor devices as they provide protection against high voltages and prevent electrical shorts between cables. Commonly used materials for this purpose include rubber, glass, and ceramics.
Copper – The Conductor of Choice
Due to its high conductivity, which is essential in high-integrated devices, copper is used in semiconductor manufacturing. Additionally, it aids in reducing propagation delay, power consumption, and enhancing circuit speed and density.
Apart from providing high thermal efficiency compared to aluminum, copper’s ductile, malleable, and highly conductive properties also contribute to its effectiveness in semiconductors. The procedure for incorporating copper into a semiconductor device involves sputtering in the initial two steps and employing a Cu electroplating method for the final step. Copper interconnects are utilized in silicon integrated circuits to minimize propagation delays.
Insulation Essentials
In semiconductor devices, insulation materials play a key role in providing electrical insulation with a high dielectric strength, preventing current flow and ensuring isolation between conductive components. This helps in preventing short circuits and maintaining the device’s electrical characteristics.
Frequently utilized insulation materials in semiconductor circuits consist of silicon-oxide dielectric and products from DuPont such as films, aramid papers, pressboard products, and thermoplastic materials. But not all insulators are created equal. Effective insulation materials for semiconductors demonstrate high resistance and hinder the flow of electricity, as exemplified by materials such as rubber, glass, and ceramics.
Inadequate insulation can result in short-circuits and breakdown of the insulation, ultimately leading to failure in power systems.
Advanced Materials for Next-Gen Semiconductors
As the semiconductor industry progresses, its range of materials also expands. Advanced materials, such as carbon nanotubes and nanomagnetic chips, are being researched for their potential to outperform traditional semiconductor materials. These futuristic materials could potentially provide a fivefold increase in energy efficiency and speed compared to silicon, in addition to advantages such as exceptional electrical conductivity, high aspect ratio, flexibility, and reduced material and energy demands.
However, the integration of these advanced materials into semiconductor manufacturing comes with its own set of challenges, including the control of the interface between these materials and metals, and the assurance of contact and CMOS process compatibility. Despite these hurdles, the potential of these materials in transforming the semiconductor industry cannot be underestimated.
Carbon Nanotubes: A Potential Silicon Successor
Considered one of the forms of carbon, carbon nanotubes (CNTs) are cylindrical structures composed of carbon atoms and characterized by their nanometer-scale diameter. These structures are considered quantum materials and demonstrate excellent electrical, mechanical, and thermal properties, making them a potential replacement for silicon in semiconductor technology.
CNTs offer superior transistor performance compared to silicon due to their ability to operate faster and at a lower supply voltage than silicon-based transistors. Their almost atomically thin structure and excellent electrical conductivity make them better semiconductors than silicon. However, working with CNTs in semiconductor production is not without its challenges, including the necessity to overcome impurities and control the interface between CNTs and metals.
The Magnetic Appeal of Nanomagnetic Chips
Nanomagnetic chips are computer chips composed of minuscule magnets that leverage magnetic properties to execute computations and process data, potentially with notable energy efficiency. This makes them suitable for applications such as:
- Artificial intelligence
- Data processing
- High-performance computing
- Internet of Things (IoT)
- Quantum computing
These chips have the potential to revolutionize the field of computing and open up new possibilities for technology.
These chips employ switchable magnetic modes such as Rashba spin-orbit torque and local magnetic fields generated by on-chip sources to manipulate data. The benefits of using nanomagnetic chips for data processing include nonvolatility, reduced power consumption, energy-efficient data processing, and the capability to facilitate the development of the internet of things and execution of artificial intelligence tasks.
Their energy efficiency is achieved through the utilization of magnetic field orientation for information representation and processing, which demands notably less energy than the electrical current utilized in silicon-based chips.
The Semiconductor Manufacturing Landscape
The landscape of semiconductor manufacturing is intricate, involving a multitude of steps, including:
- Wafer creation
- Oxidation
- Photolithography
- Etching
- Doping
- Metallization
- Packaging
The process of creating a semiconductor device, including manufacturing transistors, typically spans over a period of more than three months from design to manufacture semiconductors.
Over the years, the semiconductor manufacturing process has undergone significant evolution due to advancements in digital, analog, tools, manufacturing technologies, and materials. However, this process is not without its challenges, including:
- materials handling
- recycling
- process improvements
- time
- cost
- performance
- innovation
- supply-chain issues
Yet, in spite of these challenges, cleanrooms play a pivotal role in semiconductor manufacturing by establishing a highly regulated and sterile environment, upholding stringent cleanliness requirements imperative for the fabrication of electronic components.
The Lifecycle of a Semiconductor Wafer
The lifecycle of a semiconductor wafer involves numerous intricate steps, such as:
- Deposition
- Photoresist
- Lithography
- Etch
- Ionization
- Packaging
This process typically spans over a period of more than three months from design to production, showcasing the production capacity of the facility. It speaks volumes about the advancements in materials, fabrication, design techniques, and quality control, all leading to enhanced performance and extended lifetimes.
Factors like moisture and corrosion can impact the lifespan of a semiconductor wafer, with the reliability of these devices assessed for a period of up to 21 years when stored in a climate-controlled warehouse. At the same time, the implementation of quality control processes is crucial in the manufacturing of semiconductor wafers. These processes help improve the reliability, functionality, and overall longevity of the wafers by preventing contamination and defects that could potentially undermine the performance and reliability of the semiconductor devices.
Technological Evolution and Material Innovation
The semiconductor industry stands as a powerful example of the influence of technological evolution and material innovation. Advancements in semiconductor materials, digital and analog technologies, and manufacturing technologies have significantly influenced semiconductor technology, improving the performance, efficiency, and capabilities of electronic components. These advancements have also led to significant developments in creating the world’s first functional semiconductor using graphene.
With new materials like:
- silicon
- germanium
- gallium arsenide
- cubic boron arsenide
- aluminium nitride
- carbon nanotubes
Utilized in semiconductor fabrication, the industry continues to expand the applications of electronic devices from consumer electronics to automotive, medical devices, and other industries. Electronics manufacturers are driving continued innovation in chip technology, which is anticipated to fuel growth in areas such as 5G, artificial intelligence, and autonomous vehicles.
Sustainability in Semiconductor Production
The environmental impact of the semiconductor industry grows alongside its expansion. Therefore, sustainability in semiconductor production is of utmost importance. Strategies such as water recycling, reuse, and restoration can be utilized to reduce water usage in semiconductor manufacturing. Additionally, the implementation of water treatment and reclamation processes, such as reverse osmosis, can aid in reducing water consumption and costs.
Similarly, recycling in semiconductor production plays a crucial role by involving:
- The capture of unutilized process gases and by-products
- Reducing the need for raw materials
- Lowering the environmental impact
- Contributing to the sustainability of semiconductor production.
Summary
In conclusion, the world of semiconductors is a fascinating one, powered by a blend of science, technology, and innovation. From key materials like silicon, germanium, and gallium arsenide, to doping agents like boron and phosphorus, to metals and insulators like copper and silicon oxide, each plays a unique role in the fabrication of semiconductors. The industry also continues to explore advanced materials like carbon nanotubes and nanomagnetic chips, promising a future of even more efficient and sustainable semiconductor devices.
Frequently Asked Questions
What are the raw materials for semiconductors?
The raw materials for semiconductors include polysilicon for silicon ingot production, ultrahigh purity polysilicon for wafer slicing, and semiconductor materials such as silicon, germanium, and gallium arsenide. Germanium, one of the earliest semiconductor materials, has four valence electrons.
What is the key material for semiconductor?
The key material for semiconductors is silicon, which is widely used in producing integrated circuits (ICs) and contains multiple transistors allowing the flow of electricity to be controlled.
Who produces raw materials for semiconductors?
China is the main producer of raw materials for semiconductors, including critical minerals like REEs, Gallium, Germanium, Arsenic, and Copper, as well as raw silicon. The U.S. heavily relies on imports for these materials, with China being the dominant source.
What is the role of doping agents in semiconductors?
Doping agents, like boron and phosphorus, are purposely added to semiconductor crystals to modify their electrical conductivity and improve their performance.
How do carbon nanotubes improve the efficiency of semiconductors?
Carbon nanotubes improve the efficiency of semiconductors by offering superior performance compared to silicon, operating faster and at a lower supply voltage.